How are axolotls able to regenerate, and what blocks regeneration in us?
The Murawala lab combines diverse disciplines (developmental biology, evolutionary biology, epigenetics, genomics, and biophysics) and next-generation technologies such as gene manipulation, whole-body imaging, and -omics technologies (single-cell, single-nuc, spatial, multiome etc.), to understand the mechanisms of complex tissue regeneration.
Research Topics
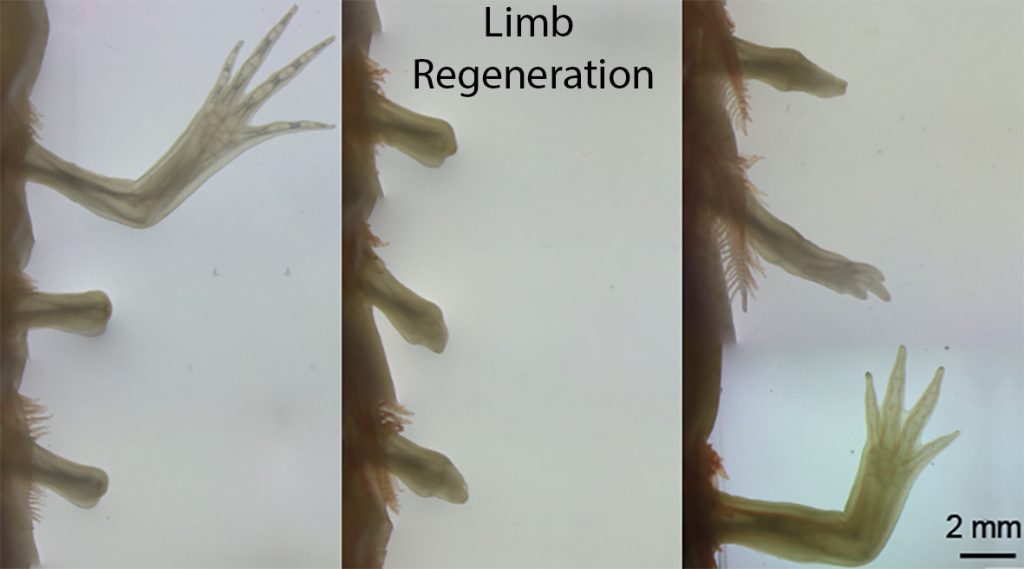
Limb regeneration
The limb is a complex structure consisting of numerous tissue types including epidermis, bones, muscles, fibroblasts, nerves, vasculature, and immune cells. Upon limb amputation, these cells coordinate with each other and carry out the extraordinary feat of precisely restoring the lost portion of limb. How do the cells know where the amputation was made? How do they know when to stop regenerating? How do they form an exact replica that contains all of the proper skeletal elements? These are just some of the questions that keep the Murawala lab busy.
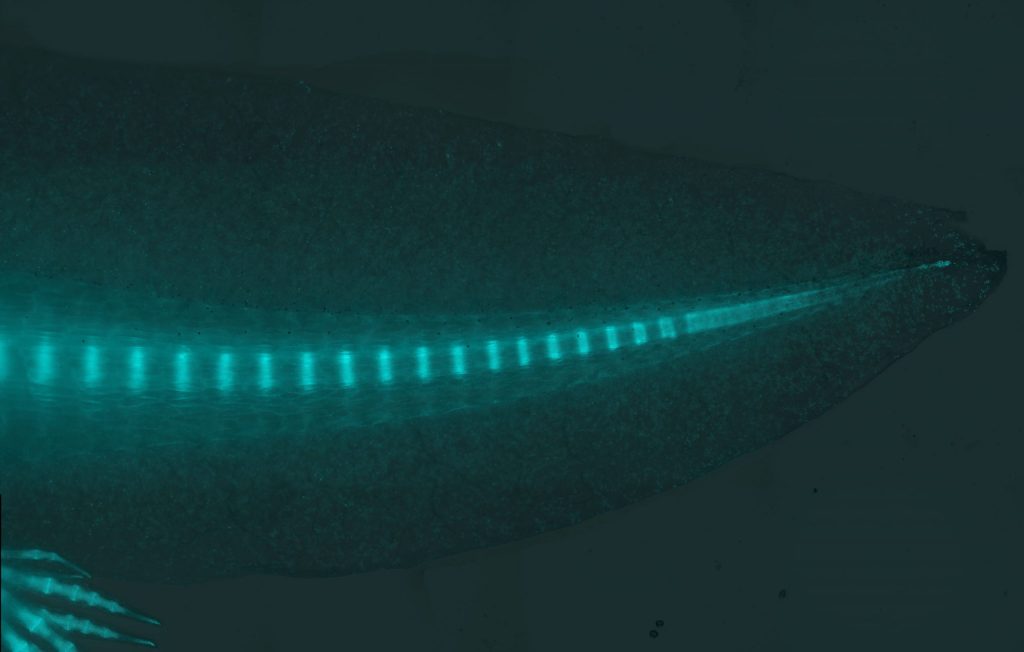
Tail regeneration
The axolotl is one of few organisms that can regenerate its primary body axis, which includes myotomes and vertebrae and extends into the tail. Interestingly, the process of myotome and vertebrae patterning during regeneration differs from that during embryonic development. During embryonic development, somitic progenitors migrate to the notochord, and through the process of re-segmentation pattern themselves into vertebrae, whereas each somite gives rise to individual myotomes. However, during tail regeneration, this patterning takes place in the absence of a notochord. As tail regeneration proceeds, a cartilage rod appears beneath the spinal cord and then self-patterns into vertebrae. At the same time, blastema cells on both sides of the spinal cord spontaneously pattern into muscle bundles. Muscle and vertebrae patterning both take place in the absence of somites during tail regeneration. We recently identified resident progenitor cells in axolotl tail that are capable of forming entire somitic mesoderm lineages including muscle and vertebrae during tail regeneration. Using-state-of-the-art technologies, the Murawala lab continues to ask, what are mechanisms underlining patterning and regeneration in axolotl tail?
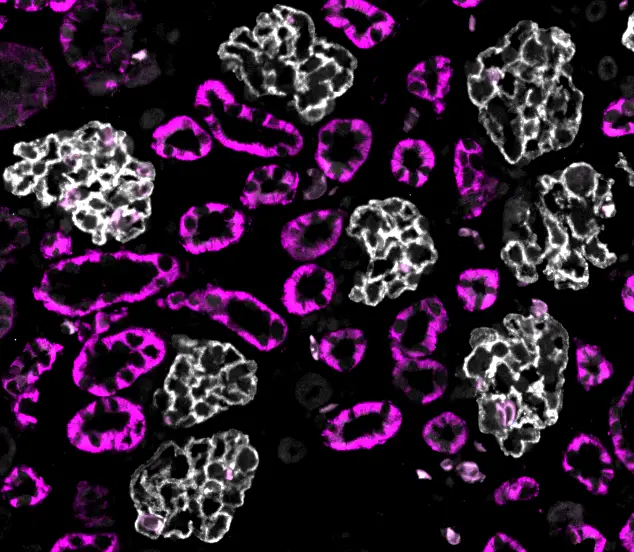
Kidney regeneration
Kidneys are vital for filtering waste and maintaining balance in the body, yet their limited ability to regenerate makes kidney disease a major clinical challenge. Our lab investigates how axolotl kidneys grow and regenerate, with a focus on post-embryonic development and injury-induced repair. We explore how new nephrons are continuously added as the animal matures, alongside how the kidney regenerates after damage. Using imaging and molecular approaches, the aim is to identify shared and distinct mechanisms driving both growth and repair. By drawing parallels between development and regeneration, this work seeks to uncover fundamental principles that may inform new strategies for treating kidney disease in humans.
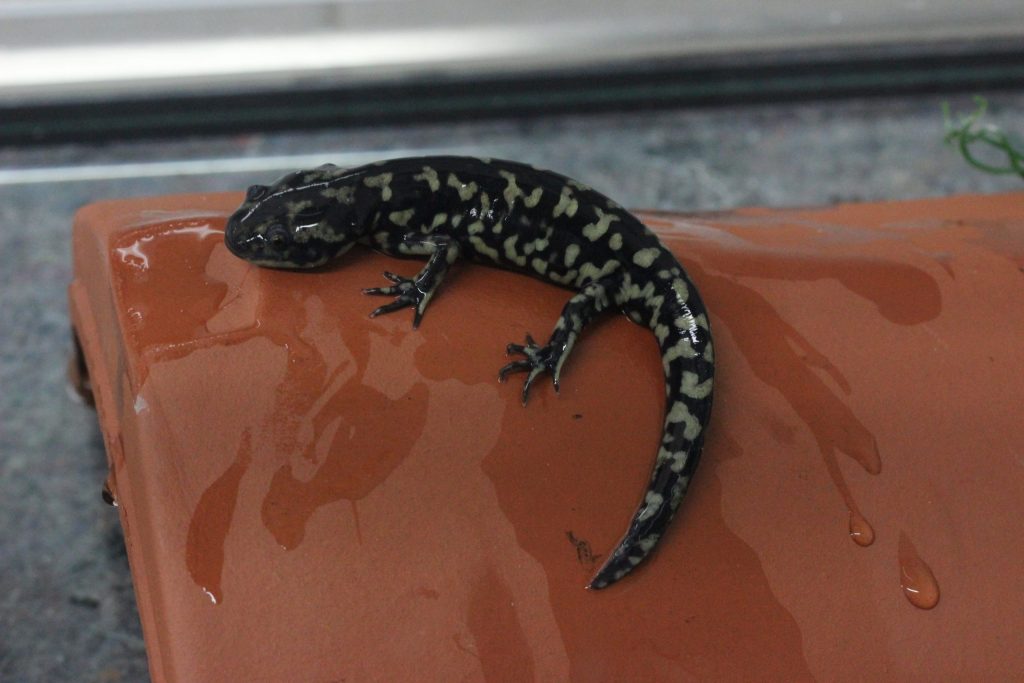
Tissue metamorphosis
Axolotls are full of wonder. Although they spend most of their life in neoteny, in the lab they are capable of metamorphosis. A single exposure to L-thyroxine initiates metamorphosis in axolotls, transforming their body. They retract their gills and start breathing with their lungs. They also shed their skin, and the new, emerging skin is more compatible with the terrestrial habitat. Axolotls also lose their fin, and the shape of their tail changes from flattened in cross-section to round. Interestingly, following metamorphosis axolotls can still regenerate some tissues, although there is a small decline in regeneration rate and fidelity. The Murawala lab wants to understand the cellular and molecular basis for metamorphosis and its implications for tissue regeneration.
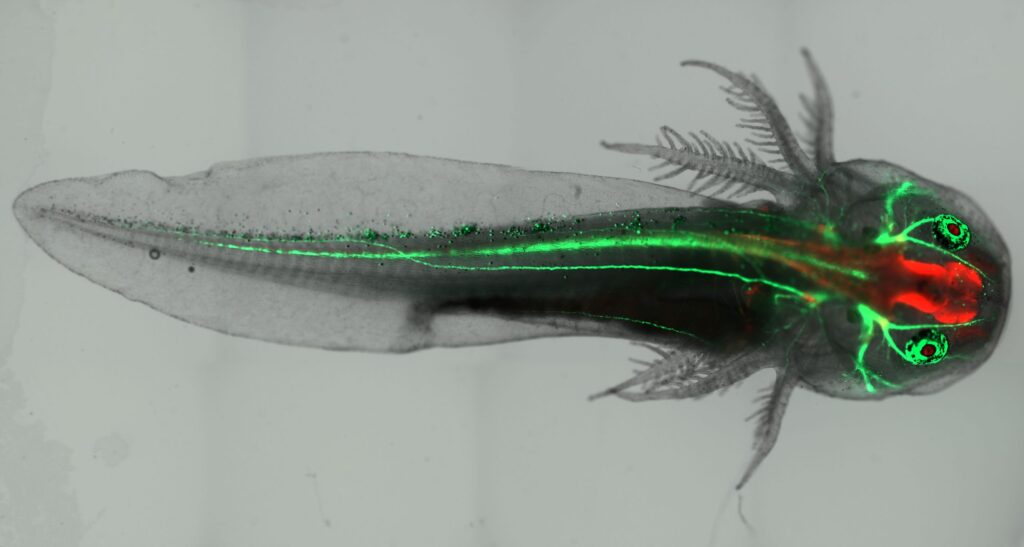
Transgenic methodologies
Genetic manipulations have completely revolutionized biological science. However, while transgenic methodologies have been widely deployed in mice, zebrafish, and Drosophila since the 1990s, their use in axolotl lagged behind. We have devoted a great deal of time and effort refining and improving these methodologies for use in axolotl, enabling us to develop I-SceI transgenesis, CRISPR-mediated knock-in and knockout, tissue-specific fluorescent reporters, and inducible Cre-loxP methodologies in axolotl. While much has been accomplished, we are continuously working to further improve these methods and develop new ones.
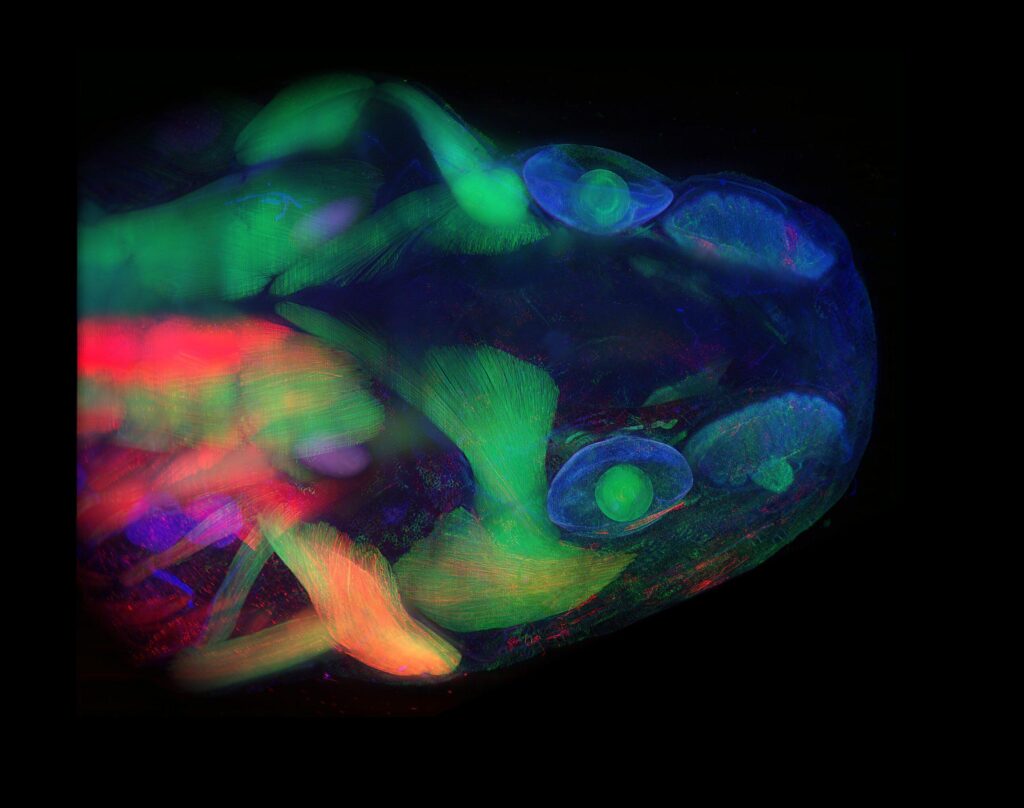
Tissue-clearing
How wonderful it would be if—when trying to investigate the internal structures of an organism—we did not have to deal with the opacity of tissues and organs. Just the thought that we might be able to see through regenerating tissue is exciting. While nature has created a few transparent organisms, most animal models are not transparent. However, by understanding the principles of light behavior and how light interacts with different substances/chemicals, we have been able to achieve a high level of transparency in fixed tissues, including tissues in whole organisms. Our lab is constantly investigating these principles and integrating our findings with state-of-the-art imaging technologies to generate high-resolution snapshots of regeneration via whole-mount imaging.
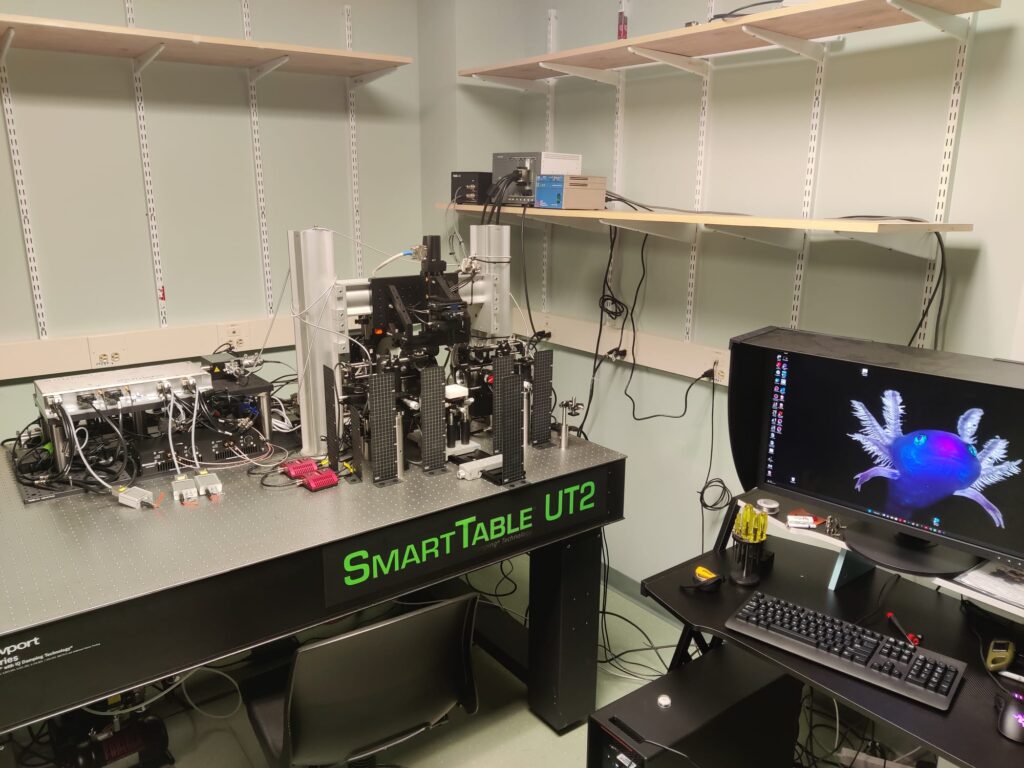
mesoSPIM microscopy
In collaboration with the developers of mesoSPIM (mesoscale selective plane illumination microscopy) imaging technologies (please see www.mesospim.org for more information), we have built a next-generation mesoSPIM microscope at MDIBL. This cutting-edge instrument, which we developed through the generous support of funds from NIGMS, enables 3D imaging of multi-cm-scale samples, including whole organisms, at an unprecedented subcellular resolution. At the time of its construction (December, 2022), this was the fourth mesoSPIM microscope on the American continent. Our mesoSPIM is under the care of MDIBL’s Light Microscopy Facility (MDIBL-LMF), which has made it accessible to researchers worldwide.